Introduction
Smartphone usage significantly impacts posture, particularly in the neck and trunk regions, leading to musculoskeletal discomfort and altered spinal kinematics. Studies have shown that smartphone addiction correlates with compromised head posture, decreased muscle endurance, and changes in neck alignment [1]. Additionally, smartphone users exhibit increased neck flexion, head flexion, and forward head posture (FHP), exceeding recommended safe angles [2, 3]. Furthermore, prolonged smartphone use is associated with neck pain, discomfort, and poor sitting neck posture [4]. This altered posture can affect the body’s centre of pressure (CoP) distribution and stabilographic variables, potentially impacting gait parameters [5]. Therefore, it is crucial to raise awareness about the ergonomic risks posed by excessive smartphone usage to mitigate musculoskeletal issues and maintain overall postural health.
Advancements in smartphone technology have led to the integration of dual-task demands, such as standing, walking, and texting or talking simultaneously [6]. Research indicates that dual-tasking with smart-phones can significantly impact gait parameters, cognitive performance, and stability [7]. Studies have shown that dual-tasking while using smartphones can increase the risk of falls due to reduced situational awareness and cognitive load [8], emphasizing the need for further examination to enhance fall-prevention interventions and community participation. Understanding the effects of smartphone-related dual-tasking on gait and cognitive performance is crucial for improving safety measures and designing interventions to mitigate potential risks associated with these everyday activities.
Maintaining a straight posture while using smart-phones affects postural control and motor performance [5, 9, 10]. Research indicates that older individuals experience a more significant decline in postural stability from smartphone use compared to younger individuals, with cognitive function deficits contributing to decreased performance during dual-tasking [11]. The complexity of postural control is further illustrated by analyzing the CoP during cognitive dual-tasks, demonstrating reduced efficacy in postural control [10]. These findings underscore the importance of considering how smartphone use impacts postural control and motor performance in everyday tasks.
Texting while walking has a more significant detrimental effect on balance, gait, and walking behaviour. Studies indicate that texting while walking leads to a reduction in gait speed, cadence, stride length, and step length, along with increased hip and ankle stiffness, which can increase the risk of fatigue [12]. Additionally, texting has been associated with a higher dual-tasking cost in terms of walking time outdoors compared to indoors, emphasizing the impact of texting on dynamic tasks in different environments [13]. Previous research has shown that texting while walking has a notable impact on balance. This is due to the dynamic nature of walking, which naturally presents challenges. However, there is no existing evidence to demonstrate that texting on a smartphone affects static balance, such as when standing on one leg. Exploring the repercussions of multitasking could greatly enrich the evidence base, aiding in understanding the mechanisms and effects of smartphone use on health, such as texting on a smartphone while balancing.
FHP significantly impacts postural stability and balance. Individuals with severe FHP exhibit impaired dynamic postural stability in the sagittal plane, which emphasizes the importance of assessing dynamic balance in FHP participants [14]. Therefore, based on the hypothesis, individuals with FHP during smartphone texting are likely to experience greater alterations in CoP during standing, particularly due to the dual-task demands, increasing their risk compared to those with normal posture. However, there has been no study comparing the effects of smartphone texting while balancing on one leg between these two groups. Previous research has shown that using a smartphone with one hand vs. two hands affects various variables. Hence, comparing the effects of using a smartphone with one hand vs. two hands on CoP during standing is of interest. The objective of this study is to compare the effects of using a smartphone with one hand vs. two hands on CoP during single-leg standing between individuals with FHP and those with normal posture. This study will offer valuable insights into the health implications of FHP during smartphone use by examining its effects on postural stability and balance, shedding light on how FHP influences musculoskeletal health and functional abilities. Investigating differences in postural control between one-handed (1H) and two-handed smartphone use (2H) will also help understand how smartphone interactions impact individuals with FHP, potentially guiding ergonomic interventions and rehabilitation programs to mitigate the negative health effects of prolonged smartphone use.
Material and methods
Study design
The present cross-sectional study employed convenience sampling methods to enrol participants, utilizing printed ads and social media platforms for recruitment. A randomized repeated design was used to investigate single-leg standing balance in three conditions: non-smartphone usage (NU; single task), 1H (dual tasks), and 2H (dual tasks), on soft and hard surfaces. The study was conducted at the Physical Therapy Laboratory, Faculty of Allied Health Sciences, University of Phayao, Thailand. CoP sway was assessed with the Nintendo Wii balance board (NWbb) for each condition while participants engaged in text typing on a smartphone and performed a single-leg standing test on both surfaces.
Participants
In the research, 57 young and healthy adults were initially part of the study. However, 7 participants were excluded for various reasons: 4 due to excess weight, 2 due to obesity, and 1 for failing the balance test during screening. As a result, the final group was composed of 50 participants aged 18 to 25 years old. All these participants were right-handed and had familiarity with smartphones for at least one year, typically spending about four hours daily on their devices. Participants with a history of trauma or musculoskeletal symptoms in the neck and upper extremities were excluded [15]. None of them had recent discomfort or trauma in the past week. The sample size calculation was conducted using the G*Power software, version 3.1.9.6 for Mac oS, incorporating mean and standard deviation values obtained from a previous investigation on postural sway [16]. An a priori power analysis was conducted to determine the required sample size using an F-test for ANoVA with repeated measures and within-between interactions. The partial η2 was set to 0.05, and the sample size was computed based on the specified alpha level, desired power, and effect size.
A power calculation was carried out with a critical α-value of 0.05, aiming for a strong 90% power level. The results indicated that a minimum of 42 participants was necessary to achieve the predetermined level of statistical significance. Prior to enrollment, all participants underwent validation to confirm the absence of any neurological or musculoskeletal disorders. They also confirmed not engaging in balance-specific training over the last six months. An assessment of limb dominance showed that all participants had dominance in their right leg, matching their dominant leg for kicking the ball [17]. After the first screening process, the participants were divided into two groups based on their craniovertebral (CV) angle: a control group (n = 25) with an angle 48° and an FHP group (n = 25) with an angle < 48° [18]. Demographic characteristics and anthropometric data are presented in Table 1. The demographic and anthropometric variables did not show any statistically significant differences (p > 0.05) between the control and FHP groups, except for the CV angle. The normal group presented a significantly higher CV angle (mean ± SD: 52.98 ± 3.54) than the FHP group (44.66 ± 2.76; p < 0.001).
Table 1
Demographic and anthropometric characteristics of the participants (n = 50)
CV angle
CV angle is measured using a method that involves photometry [19]. The CV angle is defined as the angle formed between a horizontal line through the seventh cervical vertebra (C7) and a line drawn from the tragus of the ear to the C7 vertebra. This angle is used to assess the alignment of the head and neck, particularly in relation to FHP. A smaller CV angle (< 48°) indicates a more pronounced FHP, while a larger angle (≥ 48°) indicates a more neutral head position [18]. A camera is placed 1.5 m away and adjusted to the participant’s shoulder level [19]. To ensure accuracy, markers are placed at C7 and the ear tragus. Participants are asked to stand straight, facing forward, for a lateral view photo [19]. The digital camera used was a Fujifilm (X-T100 model; 24.2 million pixels; Fujifilm corp., Japan). The images were then analyzed using Kinovea software, drawing lines between the tragus midpoint and C7 spinous process, as well as a horizontal line through C7. A CV angle below 48° indicates FHP. CV angle measurements demonstrate a high level of reliability, with a consistent test-retest reliability of 0.85–0.86 and an inter-examiner intraclass correlation coefficient of 0.88–0.89 [20].
Experimental protocol
Written consent was obtained from all participants before the study began. Participants were randomly allocated the testing sequence of six different conditions: NU, 1H (dual tasks), and 2H (dual tasks), each on soft and hard surfaces (as illustrated in Figure 1). This allocation process involved basic randomization to avoid any potential biases. During evaluations for NU, participants were asked to assess their balance on one leg while folding both hands across their chest. For smartphone usage positions, participants were instructed to maintain a neck angle of 0–15° flexion, in line with ergonomic recommendations. Shoulder angles, smartphone height, and distance were customized according to each participant’s usual smartphone habits to ensure a comfortable experience. Participants were allowed 3 min to acclimate to the measuring procedure [15]. In each smartphone usage scenario, participants were required to perform a single-leg standing test on their dominant leg while typing the English alphabet (A–Z) with their thumb, securely holding the device in their hand. Each session lasted 1 min, with a 2-min break between trials.
The single-leg standing evaluation began with participants standing with both feet on the surface. They then transferred their weight to their dominant leg as instructed, maintaining control of the smartphone and ensuring that the non-dominant knee was flexed at a 90° angle [17].
NWBB for assessment of postural sway during quiet standing
CoP was assessed using the NWBB (Nintendo, Kyoto, Japan). The NWBB is made from a plastic composite material and has four load sensors at each corner to measure vertical forces. It uses a bluetooth connection to link with a laptop computer, which is controlled by customized LabVIEW software (National Instruments, Austin, TX, USA). Data are transferred via Windows-based software designed for bluetooth. The data are sampled at 30 Hz, consistent with previous studies (10–50 Hz), and a 10 Hz cut-off filter is applied post-sampling [21, 22]. Before each test, the NWBB was calibrated with a 20.4 kg weight [23]. CoP variables such as path length sway, anteroposterior (AP) sway amplitude, and mediolateral (ML) sway amplitude were measured in centimetres.
Statistical analysis
Participant demographic data were initially summarized using descriptive statistics such as means and standard deviations. A Shapiro–Wilk test was used to assess the normal distribution of variables. The chi-square test was used to examine the differences in gender between groups. A two-way mixed ANOVA was performed to evaluate the interaction effects between conditions (NU, 1H, and 2H) and groups (FHP and normal) on CoP sway variables in all directions. Independent t-tests were conducted to compare CoP sway variables between groups under different test conditions. Within-group comparisons across conditions were performed using one-way repeated measures ANOVA, followed by Bonferroni post hoc analysis to identify pairwise differences. Data analysis was conducted using SPSS software version 26.0 (IBM SPSS Statistics, SPSS Inc., Chicago, IL, USA), with a predetermined significance level (alpha) set at 0.05.
Results
The interaction effects (group × conditions) on CoP sway were significant for all variables, including path length sway, AP sway amplitude, and ML sway amplitude, on both soft and hard surfaces (partial η2 = 0.966 to 0.988, p < 0.001). The between-group (normal vs. FHP) interaction effects were significant for all variables on both soft and hard surfaces (partial η2 = 0.187 to 0.373, p < 0.01). Additionally, the within-group (NU vs. 1H vs. 2H) interaction effects were significant for all variables on both soft and hard surfaces (partial η2 = 0.379 to 0.752, p < 0.001). Post hoc tests revealed significant differences between NU vs. 1H and NU vs. 2H, but no significant difference was found between 1H vs. 2H, as shown in Table 2.
Table 2
Two-way, mixed-effects analysis of variance of covariance with repeated measures (2 groups × 3 conditions), between-group interaction effect (normal vs. FHP), and within-group interaction effect (NU vs. 1H vs. 2H).
[i] NU/HS – non-usage on hard surface, 1H/HS – 1 hand on hard surface, 2H/HS – 2 hands on hard surface NU/SS – non-usage on soft surface, 1H/SS – 1 hand on soft surface, 2H/SS – 2 hands on soft surface * significant difference at p < 0.05, ** significant difference at p < 0.001 a significant difference between NU and 1H, b significant difference between NU and 2H
When comparing the normal and FHP groups, it was observed that the FHP group exhibited greater CoP sway length in all directions, including path length sway, AP sway amplitude, and ML sway amplitude, across all conditions: NU (single task), 1H (dual tasks), and 2H (dual tasks), on both soft and hard surfaces. These differences were statistically significant (p < 0.05), as shown in Table 3 and Figure 2.
Table 3
Comparison of CoP sway variables in each direction between FHP and normal groups, on both soft and hard surfaces (n = 50)
[i] NU/HS – non-usage on hard surface, 1H/HS – 1 hand on hard surface, 2H/HS – 2 hands on hard surface NU/SS – non-usage on soft surface, 1H/SS – 1 hand on soft surface, 2H/SS – 2 hands on soft surface FHP – forward head posture For between groups, * significant difference at p < 0.05, ** significant difference at p < 0.001 For within groups, a significant difference between NU and 1H, b significant difference between NU and 2H c significant difference between 1H and 2H
Figure 2
Comparative analysis of CoP sway between groups; the FHP group and the normal group, when utilizing a smartphone and maintaining a single-leg stance in different conditions, and within-group between three conditions; NU, 1H, and 2H on hard and soft surfaces.
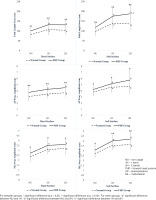
Within-group comparisons of smartphone usage revealed that both 1H and 2H smartphone usage led to a significant increase in CoP sway length compared to NU in all directions, on both soft and hard surfaces (p < 0.05), in both groups. However, no significant differences were observed between 1H and 2H, except in the FHP group, where 2H resulted in a significantly greater increase in path length sway compared to 1H on soft surfaces (p < 0.05). No such difference was observed in the normal group, as shown in Table 3 and Figure 2.
Discussion
This study is dedicated to investigating the complex interplay between smartphone use and postural stability control, with a specific focus on CoP when balancing on a single leg. The primary objective is to explore how this relationship varies between individuals with FHP and those with normal posture. It also aims to investigate the differences within each group based on diverse patterns of smartphone use, including non-usage, one-hand, and two-hand use, on various surfaces. The study aims to uncover the impact of smart-phone dependency on the musculoskeletal health of individuals with FHP by analyzing the complex interactions and emphasizing the importance of understanding how smartphones affect posture control in vulnerable groups such as those with FHP due to their widespread use in society. Additionally, the study aims to investigate how specific smartphone usage patterns affect postural stability in individuals with FHP compared to those with normal posture, potentially leading to tailored strategies to mitigate the negative musculo-skeletal effects of smartphone use, especially for high-risk groups, and bridging the gap between technology use and musculoskeletal health outcomes to inform clinical practices and public health initiatives promoting healthier smartphone behaviours and reducing hazards of prolonged use, particularly for individuals with FHP.
The findings of this study unequivocally demonstrate that when comparing individuals’ attempts to maintain balance on one leg on both hard and soft surfaces, significant differences arise between those using smartphones and those not using smartphones. Specifically, it was observed that smartphone usage led to increased CoP sway across all directions, including path length, AP sway, and ML sway. Engaging in a dual task while using smartphones, such as walking or maintaining balance, can significantly impact one’s stability and increase the risk of falls [8, 9]. When individuals divide their attention between using a smart-phone and performing a physical task simultaneously, it can lead to decreased awareness of their surroundings and compromised balance control [9, 24]. Research suggests that dual-task activities involving smartphones can interfere with postural stability by diverting attention away from maintaining proper balance and body alignment [9]. This distraction can disrupt the coordination between sensory inputs, such as visual and proprioceptive cues, which are essential for balance control [25]. As a result, individuals may exhibit altered gait patterns, reduced stability, and an increased likelihood of losing balance or falling. Moreover, prolonged use of smartphones often involves prolonged periods of static posture, such as neck flexion and decreased head stability, which can further exacerbate the risk of falls [26, 27]. Additionally, the repetitive nature of smartphone use may contribute to muscle fatigue and decreased muscle activation, further compromising balance and stability.
The findings of this study indicate that when comparing CoP sway between the FHP and normal posture groups, the FHP group exhibited a more pronounced increase in CoP sway across all conditions. This difference was evident in every direction and on both hard and soft surfaces, regardless of smartphone usage (non-usage, one-hand use, and two-hand use). The increased CoP sway observed in the FHP group, compared to the normal posture group, during smartphone use and single-leg balancing likely results from several interconnected mechanisms. FHP is characterized by a forward position of the head relative to the shoulders, which can lead to altered alignment of the cervical spine and increased muscle tension in the neck and upper back [28, 29]. This musculoskeletal imbalance can compromise the FHP group’s ability to maintain proper postural control during challenging tasks such as single-leg balancing. Proper postural control relies on the integration of sensory inputs from the visual, vestibular, and proprioceptive systems [30, 31]. Individuals with FHP may experience disruptions in sensory integration due to changes in head and neck alignment, leading to decreased proprioceptive feedback and impaired balance coordination [32–34]. The altered alignment associated with FHP can affect neuromuscular coordination patterns, particularly in the muscles of the neck, shoulders, and upper back [35]. Dysfunctional muscle recruitment and coordination may result in inefficient postural adjustments during dynamic tasks such as single-leg balancing, contributing to increased CoP sway [36].
Engaging in dual-task activities, such as using a smartphone while balancing on one leg, imposes additional cognitive demands on individuals [8]. The FHP group may have more difficulty allocating cognitive resources effectively due to their compromised musculoskeletal and sensory systems associated with their posture [37]. This increased cognitive load can further disrupt postural control mechanisms and worsen CoP sway [38]. Individuals with FHP may adopt compensatory strategies to maintain balance, such as relying more on visual feedback or muscle co-contraction. However, these strategies may be less efficient or effective compared to those employed by individuals with normal posture, leading to greater variability in CoP sway.
Furthermore, when comparing within each group across conditions of one-hand and two-hand smart-phone use, no significant differences in CoP sway were observed in any direction, except within the FHP group. Specifically, individuals with FHP exhibited an increase in CoP path length sway when using two hands compared to one hand. This discrepancy in CoP sway based on smartphone handling was not evident in the normal posture group, aligning with earlier findings of CoP sway differences between the two groups. Thus, it is emphasized that the FHP group is at higher risk of losing balance while using a smartphone than the normal posture group. The increased CoP sway seen during two-hand smartphone use compared to one-hand use in single-leg balancing among individuals with FHP involves various factors. Using a smartphone with two hands can exacerbate musculoskeletal strain in individuals with FHP, potentially causing increased muscle tension and altered posture that affect balance [39]. Two-hand smartphone interaction may require more cognitive and visual attention than one-hand use, which can detract from balance maintenance [9], especially in those with FHP who may have limited attentional resources for postural control. Individuals with FHP, who may have reduced neck mobility [40], might struggle to visually scan their surroundings or adjust their posture effectively while using a smartphone with two hands, possibly leading to compensatory movements that increase CoP sway during single-leg balancing. These results highlight the need to examine the complex relationship between smartphone use, postural control, and musculoskeletal factors, particularly in vulnerable populations such as those with FHP.
The limitations of this study include the lack of exploration into the mechanisms underlying the effects of smartphone usage on postural stability. Incorporating measures of muscle activity and sensory feedback could provide valuable insights into these mechanisms. Additionally, the absence of longitudinal follow-up hinders understanding of the long-term impacts of smart-phone usage on musculoskeletal health. Future studies should consider longitudinal assessments to provide further insight into the effects of smartphone usage over time, thereby informing preventive strategies and interventions.
Conclusions
In conclusion, our study highlights that individuals with FHP face an elevated risk of losing balance during non-smartphone activities, such as single task, compared to those with normal posture. Furthermore, this risk of balance loss becomes more pronounced when engaging in smartphone use while maintaining single-leg standing balance (a dual task), as evidenced by increased CoP sway compared to the normal posture group. Notably, the risk is particularly heightened during two-hand smartphone use. These findings underscore the importance of considering the impact of FHP on balance control, especially in the context of smart-phone usage, and emphasize the need for targeted interventions to mitigate these associated risks.