Introduction
The hurdles event is an example of a repetitive activity with significant spatial and temporal imperatives, in contrast to the flat sprint, where the stride structure is not subject to strong external limits in terms of length or frequency [1]. A mix of many different running and jumping kinematics is required for proper hurdling technique [2]. The hurdle ubication makes it possible to divide the event into three phases: (1) the approach run phase, which extends from the starting line to the first hurdle; (2) the hurdle unit phase, which includes running between hurdles and overcoming hurdles; and (3) the acceleration phase, extending from the last hurdle to the finish line. Temporal analyses typically relate to the intermediate times between each passage of a hurdle (landing on foot). In temporal studies, referring to the flight time over the hurdle (from take-off to landing) was also frequent [3]. Tsiokanos et al. [4] noted a significant relationship between the intermediate times and final performance over 110-m hurdles (r = 0.77 to 0.98). These authors also showed that this relationship was decisive from the fifth hurdle onwards in the 110-m obstacle event (r = 0.77 to 0.98). A strong correlation (r = 0.82) was also noted between the mean flight time of the five hurdle steps and the official time [5].
The high hurdles sprint is the most demanding of the three outdoor hurdles events currently being run at the international level [6]. Thus, athletes, coaches, and researchers have sought to improve hurdle performance by placing more emphasis on the kinematic analysis of hurdling techniques. over the years, there has been a considerable amount of biomechanical literature concerning the kinematic analysis of hurdle clearance at different levels of performance in both male and female athletes [2, 7-12]. However, there is a scarcity of data that compares the distinct movement characteristics exhibited by elite hurdlers in contrast to elite athletes participating in combined events. Such a comparison is likely to highlight the factors that significantly affect performance in the 110-m hurdles, including clearance times, horizontal velocity, split times, etc., thus providing coaches with a better technique that could improve their athletes’ performance [13]. Indeed, 110-m hurdles specialists are highly trained athletes in this event and their technical gaps are minimised at all levels of ability. Decathletes are also well trained at this event but to a lesser extent than the specialists, and they are very likely to have many more deficiencies than the specialists, resulting in significantly different performance. To be a champion in all ten events, a decathlete does not necessarily have to possess exceptional qualities in just one event. They must demonstrate a minimum level of competence in their lowest-performing events while demonstrating exceptional skills in their highest-performing events. Throughout the training program, the decathlete must improve their technique, develop their strength while maintaining optimum speed and agility, and cultivate the endurance needed to endure demanding competitions, which often last 8 to 10 hours a day. Thus, the comparison between specialists and decathletes could reduce the number of parameters cited by Tsiokanos et al. [4] as direct performance descriptors and highlight the main factors that most influence performance in this discipline.
Moreover, analysis of the specialised literature shows that most studies related to the technical aspects of hurdles and sprint observations are performed during training conditions or are derived primarily from simulated events rather than from genuine competitions [14]. It is, however, difficult to effectively simulate competitive race conditions. It is also likely that athletes concentrate more fully and thus run slightly faster at an official event than under practice or other conditions [12]. This may be due to factors such as adrenaline and the fact that, in a meet, the race has to be run only once. Therefore, this study aims to establish the main factors of hurdle clearance technique efficiency by revealing, based on a comparative kinematics analysis, some important biomechanical parameters involved in the hurdle sprint techniques. The current study also aims to explore the relationship between the biomechanical characteristics of hurdle specialists and decathletes and their performance in the 110-metre hurdles. The following hypotheses were formulated: (1) elite hurdlers exhibit a higher crossing technique compared to elite decathletes; (2) elite decathletes display greater vertical displacement during flight than hurdlers; and (3) reduced contact time during the take-off phase and quicker speed recovery between hurdles result in minimal speed loss and better performance.
Materials and methods
Participants
Using G*Power version 3.1.9.7 (Heinrich Heine University Duesseldorf, Duesseldorf, Germany) and referring to the method described by Howell [15], a minimum of 16 participants, with 8 subjects per group, should be tested to have a significant difference between the mean performances of two groups in the 110-metre hurdles (based on preliminary data) while assuming a large effect size, a probable error of 0.042 and a power (1- probable error) of 0.95. Keeping in mind a dropout rate of 20%, as reported by bell et al. [16], a sample of 20 elite athletes was selected for potential participation in the study. These were 10 male elite hurdles specialists [(EHS), (age: 20.9 ± 2.2 years, body mass: 76.9 ± 7.0 kg, height: 1.85 m ± 0.05 m)], and 10 male elite decathletes [(ED), (age: 20.8 ± 2.27 years, body mass: 87.7 ± 6.9 kg, height: 1.91 m ± 0.03 m)]. All participants were affiliated with the Tunisian national track and field team and had a minimum of five years of experience in their respective areas of specialisation. Additionally, they often took part in national and international competitions, but unfortunately, none of them made it to Tokyo for the 2020 Summer olympics. The protocol was carried out following the latest Declaration of Helsinki [17], all procedures were approved by the Institutional review Committee for the ethical use of human subjects at the Higher Institute of Sports and Physical Education in Ksar-Said, Tunisia, and the authorisation to perform the necessary measurements was obtained from the technical committee of the Tunisian Athletics Federation. All participants were informed of the experimental protocol and its potential risks and benefits, duly signed an informed consent form, and were permitted to withdraw from the study at any time and without explanation.
Procedures
The experimental procedure was implemented during a series of meetings held by the Tunisian athletics team on three distinct occasions, with the sequence of these meetings being randomised. The participants included in the training session consisted of both hurdlers and decathletes and each ran the 110-metre hurdles four times over a minimum of two meetings, with at least an hour elapsed between each pair of trials. Every attempt was executed by a duo of athletes in a way closely resembling a genuine competition, with each athlete being recorded on two occasions and the best performance was retained for statistical analyses. The athlete chosen for kinematic analysis was strategically positioned on the right-hand side, offering the best view of the field of vision of two synchronised cameras. The first camera was positioned at hurdle level in a plane perpendicular to the direction of the athlete’s movement, while the second camera was positioned at the edge of the running track, at right angles to the first (Figure 1). This arrangement captured various body parts from different angles. The experimental procedures were conducted under warm temperature conditions, specifically within the range of 26–27°C, with a wind speed below 1.0 m/s.
Motion analysis
Kinematic analysis of the 110 m hurdles sprint was performed for each athlete at the 5th hurdle. Two synchronised high-speed cameras (Arriflex Sr-II, Germany) equipped with telephoto lenses (Angenieux 10–150 mm, France) and operating at 100 Hz were used to establish the kinematic parameters [18]. Targets were temporarily placed on the track to calibrate the space volume travelled by the hurdler, to ensure it was sufficiently large to contain the hurdler. The location and height of the cameras made it possible to film the entire hurdle-crossing phase and the complete cycle of the first stride after the filmed hurdle. In the analysis of the hurdle-clearing phase, three phases were examined: (1) the take-off phase, which refers to the final braking and propulsion actions before clearing the hurdle, (2) the flight phase, which pertains to the duration of time spent in the air and the clearance achieved above the hurdle, and (3) the landing phase, which encompasses the initial braking and propulsion actions following the successful clearance of the hurdle.
Twenty adhesive reflective markers were placed on each participant’s body to digitise the clearance data for kinematic athletes. The markers were strategically placed on the cranium area, the thorax, and the axes of rotation of the arm and leg joints, ensuring that each mark was always detectable by at least one camera. The video recordings were then transferred to a laptop and the body indicators were digitised using the video data analysis program SkillSpector® 1.3.2 (Video-4coach, odense, Denmark). SkillSpector makes it possible to calibrate the cameras using a calibration frame and applying the Direct Linear Transformation (DLT) algorithm. The whole process was supervised by the same person, an expert in motion analysis, and the results were approved by the authors.
The various segments of the body were considered as non-deformable solids interconnected by perfect connections. The landmarks used were the endpoints of the 14 segments into which the body was divided (head, trunk, right hand, left hand, right upper arm, left upper arm, right forearm, left forearm, right upper leg, left upper leg, right lower leg, left lower leg, right foot, and left foot). The segments’ inertia was determined based on Hanavan’s model [19], which considers the segments as truncated cones (feet, legs, thighs, arms, forearms, and hands) or revolution truncated ellipsoids (pelvis, trunk, head, and neck). The three-dimensional (3D) coordinate positions were precisely known by the positions of targets placed temporarily on the runway to calibrate the volume of the runway evolution surface. Such calibration makes it possible to locate the orthogonal reference system (r) from which the projection coordinates relative to the various body segments will be carried out. 3D coordinates of the segmental body joint data were collected using motion analysis software (regavi & regressi, Micrelec, Coulommiers, France). The video sequences were stripped image by image, indicating the successive positions of each segment joint during movement. We reconstituted the body segments considered rigid to make kinograms of each hurdler. The centre of mass (CM) of the body segments was calculated using the Hanavan model adjusted by De Leva [20], and the joint angles were defined as relative to their adjunct body segments. For example, the vector connecting the elbow and shoulder reference points (V1) constitutes the orientation of the upper arm, while the vector connecting the elbow and wrist reference points (V2) constitutes the orientation of the forearm. The angle between these two vectors constitutes the elbow angle [21]. Selected kinematic parameters were calculated using scientific graphing and data analysis software (SigmaPlot 10.0, Systat Software Inc., USA). For smoothing, we used a second-order low-pass filter with an 8-Hz cut-off frequency determined from a residual analysis [22].
A multitude of spatial and temporal data were acquired from the digitised files. Prior to the hurdle, the take-off distance was delineated as the horizontal span extending from the metatarsophalangeal joint, which signifies the foot region in closest proximity to the ground, at the precise instant of take-off, to the foundation of the hurdle (Figure 1). The terms ‘CM distance’ and ‘height’ were utilised to describe the horizontal and vertical positions, respectively, of the centre of mass in relation to the metatarsophalangeal joint during touchdown and take-off, both prior to and subsequent to clearing the hurdle. Following the successful completion of the hurdle clearance, the landing distance was subsequently determined as the horizontal measurement between the metatarsophalangeal joint and the hurdle. The landing step length refers to the horizontal distance travelled by the initial step taken after clearing the hurdle, measured from the point of contact to the opposite side’s point of contact. The knee angle refers to the angle in the sagittal plane, which is determined by the positions of the hip, knee, and ankle joint centres. The ankle angle can be described as the angle inside the sagittal plane, which is determined by the positions of the knee, ankle, and metatarsophalangeal joints [23].
Statistical procedures
The normality of all distributions was assessed using the Shapiro-Wilk test, and any distributions that did not fit the normal distribution were subjected to a log transformation. A paired sample t-test was computed to assess any systematic bias in the data collection between the two 110-m hurdle race performances. To determine whether there were significant differences between the two performances in the 110 m hurdles, a paired-sample t-test was used. The intra-class correlation coefficient, or ICC, was computed as an index of relative reliability to look at how well the participants held their positions throughout trials. The typical error of measurement, or TEM, was calculated to evaluate the amount of variation in repeated measurements for participants (absolute reliability) [22]. We defined an ICC of less than 0.50 as poor, 0.50 to 0.75 as moderate, 0.75 to 0.90 as acceptable, and > 0.90 as excellent [24]. Cohen’s d was determined using an online calculator developed by Lenhard and Lenhard [25] for the computation of different effect sizes (ESs). d was interpreted using the following scale: 0.2 (trivial); 0.2–0.6 (small); 0.6–1.2 (moderate); 1.2–2.0 (large); and > 2.0 (very large) [26]. The differences between groups (EHS vs ED) were assessed using Student’s t-test, and the Pearson correlation coefficient was used to examine the association between the performance across 110-m hurdles (dependent variable; DV) and the spatial and temporal characteristics (independent variables; IV). In addition, the linearity of the IV was tested using scatter plots. The normality and homoscedasticity of the residuals were also verified, and the IV variables were checked for multicollinearity using the variance inflation factor (VIF). Values were more than 10 indicating the occurrence of high intercorrelations among the IV. To address multicollinearity, a principal component analysis (PCA) was used to reduce the number of variables while retaining a significant percentage of the information contained in the data. A sequential multiple regression (SMr) approach was then used to determine the extent to which the components produced by the PCA analysis could explain the performance variance in the 110 m hurdles [27]. Threshold values for interpreting the adjusted R2 as the effect size were set at 0.02 (small), 0.13 (medium), and 0.26 (large) by Cohen [28]. Statistical analysis was performed with the SPSS statistical package (version 26 for Windows; SPSS Inc., Chicago, IL, USA), results are presented as mean ± standard deviation (SD), and the statistical significance was set at α= 0.05.
Ethical approval
The research related to human use has complied with all the relevant national regulations and institutional policies, has followed the tenets of the Declaration of Helsinki, and has been approved by the local ethics committee of the Tunisian Athletics Federation, Tunisia (registration No.: ref. 1145, october 10, 2022).
Results
Between-trials reliability
Table 1 shows the results of the reliability analysis of the data collected using the test-retest approach. There was no statistically significant difference (p > 0.05) between the two races in terms of the 110-metre hurdle clearance and the first stride post-hurdle. Absolute and relative reliability levels ranging from acceptable to excellent were demonstrated by the spatial and temporal parameters measured (ICC > 0.75, TEM < 5%).
Table 1
Data collection accuracy
Spatial and temporal data
Performances of the 110-m hurdles sprint achieved by the elite hurdlers were significantly better than those of the elite decathletes (p = 0.001, with very large ES). The average times recorded were 14.23 ± 0.36 s and 15.47 ± 0.26 s, respectively (Table 2). The comparison of the kinematic parameters between the ED and EHS revealed that the amplitude of the stride over the hurdle was significantly greater in the decathletes. This larger hurdle step length coincided with a greater take-off distance. This was clearly expressed in the attack area. The distance from the take-off point to the hurdle was 2.16 ± 0.13 m in ED and 1.95 ± 0.19 m in EHS (p = 0.002, with large ES). In contrast, the distance from the hurdle to the touchdown point, after clearance, was not different between the ED and EHS. The average hurdle stride was 3.60 ± 0.17 m for the EHS and 3.84 ± 0.27 m for the ED. The take-off distance was 1.95 ± 0.19 m and 2.16 ± 0.13 m, respectively, which represents 54.2% and 56.3% of the total hurdle stride length. The landing distance was 1.65 ± 0.16 m for the EHS and 1.68 ± 0.23 m for the ED, which was between 45.8% and 43.8% of the total hurdle stride length (see Figure 2).
Table 2
Spatial and temporal parameters of hurdle clearance and the first stride post-hurdle in Tunisian elite hurdles specialists and elite decathletes
Figure 2
Visual representation of take-off and landing distance, hurdle clearance, length of landing and the first stride post-hurdle, and the height of the CM at take-off, obstacle clearance, and on landing for elite hurdles specialists (upper) and elite decathletes (bottom). results are presented as means and the hurdle is shown as the athletes would approach it, running from left to right
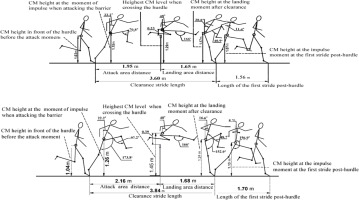
As such, the EHS were characterised by a less extensive hurdle clearance stride (p = 0.002, with moderate ES), a shorter flight time over the hurdle (p = 0.001, with large ES), and a smaller support phase of the first step inter-hurdle (p = 0.005, with large ES). Whereas, except for the moment of the supporting leg’s contact with the ground before attacking the hurdle, the CM’s path for the ED was higher than that for the EHS (p = 0.001, with large ES). The position of the CM above the hurdle was also higher for the ED (39 ± 4 cm) than for the EHS (23 ± 3 cm) (see Figure 3). The intercorrelations study showed that both groups’ performance in the 110-m hurdles was positively correlated with the time of hurdle crossing (EHS: r = 0.74, p = 0.013; ED: r = 0.63, p = 0.02; moderate ESs) and with the duration of the support phase of the first step post-hurdle (EHS: r = 0.91, p = 0.001; ED: r = 0.73, p = 0.013; moderate ESs).
Figure 3
Visual representation of the height of the CM at take-off, obstacle clearance, landing, and the first stride post-hurdle in elite hurdles specialists (■) and elite decathletes (□). results are presented as means and the hurdle is shown as the athletes would approach it, running from left to right
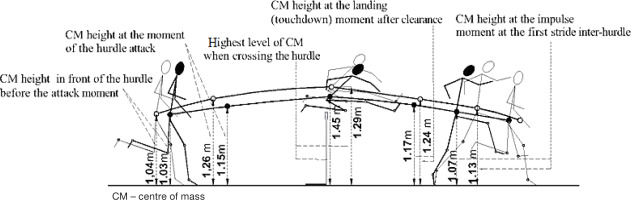
Angular kinematics
At the hurdle attack, no significant differences were observed between the two groups in terms of angulation of the supporting leg, the hip attack leg, the body tilt, or the leg impulse (Figure 4). However, at the hurdle-crossing, the EHS showed a higher degree of lead leg knee flexion than the ED (p = 0.001, with very large ES). During this phase, the tilt of the trunk forward significantly influenced the achieved performance (EHS: r = –0.70; p = 0.012; ED: r = –0.63; p = 0.02; moderate ESs). However, at the landing phase, the EHS touched down with the trail leg more flexed at the hip level, the trunk more sloped forward by a return to vertical, and a smaller positioning angle of the trailing leg (p = 0.05, with moderate ES). During this phase, the performance of the EHS on the 110 m hurdles sprint was positively correlated with the hip opening of the support leg (r = 0.66, p = 0.019, with moderate ES). Nevertheless, the sequence of the first stride inter-hurdle was made with no significant angular difference between all participants, except that of the impulsion, which was significantly more important in the EHS (p = 0.021, with large ES).
Associations between performance over 110-metre hurdles and spatial and temporal variables
The partial component analysis was carried out with 1 as the minimum eigenvalue of the factors. After Varimax rotation with Kaiser normalisation, a two-factor solution was extracted (see Table 3). The percentage of variance accounted for was 93.384%, with 47.421% attributed to factor 1 and 45.963% attributed to factor 2. The factors and sports specialty were entered into the equation one at a time to determine the contribution of each to the regression equation. As shown in Table 4, factor 1 was not correlated to performance and sports specialty, and its effect on the performance was not significant (R2 = 0.072). Conversely, factor 2 and athletic specialty significantly affected the performance achieved over the 110-m hurdles; this effect was large for factor 2 (R2 = 0.848) and small for the specialty (R2 = 0.03). A significant correlation was also noted between factor 2, sport specialty, and performance (r = 0.895 and 0.731, respectively). Factor 2 includes stride length; the flight phase time; distance: take-off point – hurdle; the amplitude and support time of the first stride after the hurdle; the height of CM at the impulsion before the hurdle, above the hurdle, at landing after clearance and at impulse at the first stride after the hurdle; the knee angle of the attacking leg in the attack phase; the hip angle and the positioning angle of the supporting leg at the landing phase; and the inclination of the trunk from the vertical at the landing phase.
Table 3
Factors extracted, eigenvalues, and proportion of variance after rotation
Table 4
Sequential multiple regression models for associations between performance over 110-m hurdles and spatial and temporal variables during hurdle clearance and the first stride after hurdles
Discussion
The current investigation conducted a comparative analysis of spatial and temporal data pertaining to running performance, specifically focusing on male athletes specialising in the 110-metre hurdles discipline and those participating in decathlon events. The relationship between spatial and temporal variables and 110-metre hurdle performance was also investigated in order to examine the running behaviour of the two types of athletes and estimate the impact of predicting characteristics on performance.
The key-results of our study indicate that elite hurdles specialists exhibit distinct characteristics when compared to elite decathletes. These include a shorter flight time, a shorter stride length over the hurdle, a lower centre of mass path, a more forward-tilted trunk during the landing phase after clearance, a shorter braking phase upon landing, a more open propulsion angle at the attack moment in front of the hurdle and at the first stride inter-hurdle, and a shorter support time during the recovery stride after clearance. Additionally, our research results suggest that factor 2, as determined by PCA, and athlete specialty have a significant impact on performance outcomes in the specific setting of the 110-m hurdles.
The analysis of the data indicates that the race performances of our 110-metre hurdles were inferior to those of all finalists in the previous olympic Games, namely Athens 2004 (13.30 ± 0.26), beijing 2008 (13.33 ± 0.25), London 2012 (13.26 ± 0.23), and rio 2016 (13.27 ± 0.13). This finding provides a rationale for the absence of Tunisian male athletes in the 110-metre hurdles event across all olympic Games. Nevertheless, the running performance exhibited by the decathletes fell within the spectrum of the most exceptional performances achieved by the top 21 participants in the men’s decathlon event during the 2020 Summer olympics [29]. These performances ranged from 3.46 to 16.10 s. The results of this study also indicate that improved hurdle sprint performance is achieved by reducing the spatial and temporal parameters of the clearing phase and the first step between hurdles. A clear difference was observed between the times for crossing the fifth hurdle (in seconds) of our participants and those of the male 110-m hurdlers at the 2004 (0.33 ± 0.01) and 2008 (0.33 ± 0.04) olympic Games [4]. This result confirms the findings of Graubner and Nixdorf [30], who identified a significant correlation between the kinematic characteristics of the compensation phase and the final performance. In contrast to the findings of Tsiokanos et al. [4], the current study demonstrates that improved performance in the 110-m hurdles sprint event depends on several technical and biomechanical parameters called direct performance descriptors, including clearance times, horizontal speed, split timing, etc., and are used to plan an athlete’s distribution of effort during competition. In the support phase, bubanj et al. [31] affirmed that the vertical force components are crucial, especially while crossing each hurdle. During the hurdle clearance, invariable and common characteristics can be distinguished. These distinctions are essential: both for determining universal mechanisms in the organisation of hurdlers’ temporal and spatial movements and for solving problems related to the presence of the obstacle [32]. This is of particular importance in combined athletics events, since the participants are obliged to develop their technical skills in several athletic specialties at the same time. In contrast, the training efforts of hurdlers are focused on improving hurdling skills, refining hurdle sprint mechanics, and establishing general sprint conditioning, as well as on the even distribution of energy throughout the race. The resolution of such tasks can be expected to be even more successful when the general mechanisms constituting the basis of such combined events, or distinct technical elements of the same discipline, have been studied appropriately.
Our results also highlight the importance of increasing the sprint rhythm to improve 110-m sprint hurdlers’ performance. This means that the velocity of running between and over the hurdles needs to be matched with the technique and agility of the runner. Agility itself depends on several factors, especially those that describe the attacking phase in front of the hurdle, the CM path, and the landing after the flight over the hurdle [31]. running speed could also be increased by optimising the support and flight time and reducing the amortisation time [13, 32–34].
The present study confirms that the EHS were distinguished from the ED by their shorter stride length over the hurdle, lower CM path, and faster crossing technique. Čoh et al. [35] found that elite athletes with shorter hurdle clearance times had faster hurdle running times. According to McDonald and Dapena [10], the criterion of an efficient hurdle clearance technique is the shortest possible time of the flight phase (hurdle clearance time); otherwise, the hurdler loses velocity in the air. According to bubanj et al. [31], this condition is a very effective way to reduce the vertical oscillations of the CM, since it allows the athlete to maintain a stable horizontal velocity and develop a consistent stride pattern. our results, determined under experimental competitive conditions, were in accordance with the findings of Hanley et al. [23], who performed a competition analysis of eight finalists from the men’s 110 m hurdles at the London 2017 World Championships. our findings further reveal significant differences between the ED and EHS in the first stride post-hurdle that are related to the latter’s superior performance. This could be explained by the necessity to minimise the braking forces to maintain a constant horizontal velocity along the race and enable a quick resumption of optimal speed between the hurdles [5]. Indeed, it is widely accepted that one of the most important characteristics of the effectiveness of the hurdle sprint techniques is the ability to maintain a high and constant running rhythm without losing balance, especially after crossing the hurdle at the touchdown moment [36], and that the braking phase must be as short as possible [2]. Moreover, a typical technical fault, particularly among novice runners, is the considerable loss of speed after the touchdown, which leads to a substantial reduction of the horizontal velocity and amplitude of the first stride after the hurdle [8, 28]. As such, it becomes clear that an optimal flight time in the EHS during the recovery stride (post-hurdle) reflects a more efficient running technique [37]. This underlines the finding of Li and Fu [38] that the first stride after the hurdle is a crucial factor that determines whether the hurdle clearance technique is satisfactory.
The comparative analysis of the angular kinematics reveals that the EHS are distinguished by a more pronounced forward slope of the trunk at hurdle-crossing, particularly during the landing phase. This body posture at the landing phase creates auspicious conditions for an active landing after hurdle-crossing, minimising the loss of speed mainly during the amortisation phase, ensuring easier forward locomotion [35], and allowing the CM to lower to an optimal level above the hurdle [2]. It should also be noted that the EHS show more efficient movement of the attacking leg at the moment of propulsion and hurdle attack. The lead leg’s smaller knee angle reduces its moment of inertia at the attack of an obstacle [31]. According to Čoh et al. [2], the leading leg increases the value of the horizontal CM velocity of the athlete at hurdle-crossing.
Limitations
Although the present investigation possesses several notable merits, it is imperative to acknowledge that it is also subject to certain limitations. Initially, the measurements were obtained within a simulated measuring scenario that emulated an official competitive environment, rather than being derived from an actual competition. Fernández-Fernández et al. [39] found that athletes had more pronounced psychophysiological reactions throughout the day when exposed to real-world competitive contexts. Moreover, there was a stronger correlation observed between hormone levels, self-reported stress levels, and workload during actual competition compared to training sessions. The athlete received vocal encouragement during the duration of the exercise, while the coaching staff imparted precise instructions in order to challenge the athlete’s boundaries. Furthermore, within the framework of this research, it would be more suitable to employ a greater quantity of synchronised cameras (specifically, 6 or 12 cameras) strategically positioned at various locations to adequately capture the subtle intricacies of performance related to the 110-metre hurdles, thus reducing the potential error arising from utilising only two cameras. Nevertheless, doing the experiment outdoors at the racing track rather than in a sports hall presents challenges in utilising a substantial number of cameras. In this scenario, employing two synchronised cameras seems to be adequate for carrying out the research [40]. The study’s findings may have been greatly impacted by the anatomical characteristics of the individual ED and EHS. Hence, it is imperative to augment the current investigation by incorporating more studies that examine the influence of these characteristics on the performance outcomes of the 110-m hurdles.
Conclusions
The comparative kinematic examination of the EHS and ED’s fifth hurdle clearance and the subsequent first stride post-hurdle identified the common components and specific distinguishing technical characteristics of each group. Some of the most essential factors have been determined by evaluating the kinematic peculiarities throughout the launch, flying, and landing phases. It was discovered that the EHS differed from the ED by having a shorter flight time, a shorter stride length over the hurdle, a lower CM path, a more forward-tilted trunk at the landing phase after clearance, a shorter braking phase at landing, a more open propulsion angle at the attack moment in front of the hurdle and at the first stride inter-hurdle, and a shorter support time at the first stride after clearance. our findings also revealed that the EHS were defined by more reasonable and efficient hurdling strategies, which resulted in superior outcomes than the ED. The results also revealed that factor 2 had a significant impact on performance over the 110-m hurdles. The athletic specialty had a minor but important impact on performance. The data generated by the EHS and ED during the 110-m hurdles sprint during an official competition simulation could be valuable for three reasons: first, to estimate hurdling techniques, second, to gain a better understanding of hurdling-specific parameters and third, to contribute to a baseline of the differences between the measured variables between practice sessions and real events. We believe that with this insight, hurdling strategies might be tailored individually for each event rather than in general, perhaps leading to higher performance.
Practical implications
The current findings underscore strategies for mitigating the decline in velocity, taking into account the constraints of our study. In order to achieve ideal velocities between hurdles, sprint hurdlers must ensure they maintain an efficient sprinting mechanism. This can be achieved by adhering to the following guidelines:
During the take-off phase, hurdlers should aim for a more open propulsion angle. This means that the angle at which they push off the ground should be wider, ensuring a more effective transfer of energy [41].
In the flight phase, hurdlers should strive for a shorter flight time, shorter stride length over the hurdle, and a lower centre of mass trajectory. These adjustments contribute to a more efficient and controlled movement over the hurdle [2].
On landing, hurdlers should adopt a more forward-leaning trunk position and minimise the duration of the braking phase. This posture helps to maintain momentum and reduce the deceleration caused by excessive braking [42].
Finally, hurdlers should focus on achieving a more open propulsion angle and shorter stance time on the first stride after clearing the hurdle. This facilitates a quick and powerful start to the next phase of the race. by adhering to these principles, hurdlers can enhance their speed and efficiency in hurdling events [23].